“Big Bang” : A terrible name for a great theory [from the archives]
Posted on August 21st, 2009 — permalink
[This was originally posted in June of 2006 on an earlier incarnation of this blog that's no longer available. It was collected in "The Open Laboratory: The Best Writing on Science Blogs 2006" I'm reposting it here so that it will again be available to the broader net.]
Perhaps, from a marketing point of view, “Big Bang” is a great name. It’s short, it’s punchy, it evokes memorable images, and it’s easy to remember. But, marketing is something I really don’t understand; as we all know, it’s easy to fear what we don’t understand (particularly when it has a huge amount of control over us and the world around us), and to hate what we fear, and as such I’m very convinced in my opinion that Marketing is Evil.
So why is the Big Bang a terrible name? Because the name itself evokes and supports many misconceptions about the theory. Read on for my musings on the matter.
The Big Bang was not an explosion.
The name suggests an explosion: some point that blew up, and everything outside it is now rushing away from it due to the force of the explosion. It’s an analogy that does provide some useful intuition (without Dark Energy, the Universe is expanding because of the left over expansion that started way back when and hasn’t been stopped by gravity), but there are a lot of problems with it. Most notably, galaxies are not in fact flying away from each other! A much more modern way to look at it, and a way that matches the mathematics that physicists and astronomers really use when they work with the model of the expanding Universe, is that galaxies (except for little local motions) are pretty much fixed, but space itself expands. Galaxies get farther apart from each other because, as space expands, there’s more space between them. (Note that the galaxies themselves are not expanding; they are held together by their own forces.) An analogy is rising raisin bread: the bread expands, and the raisins get farther apart, but the raisins aren’t moving through the bread. Another analogy would be pennies pasted on the surface of a balloon.
[Addendum added 2009-08-21: there is some debate in the astronomical community as to whether "galaxies flying apart" or "space expands" is a better qualitative description of the mathematics of the Big Bang. Obviously, I'm in the "space expands" camp. I'll write more about this in a later post.]
The Universe didn’t all start from one point.
Sometimes you will hear people say this. The classical Big Bang model (but see below) has an initial singularity; at that beginning, any two points which are separated by a finite distance now were separated by zero distance. That sure seems like things begin at an initial point. It is, however, much better to say that the initial singularity is a point where our models break down, and that all we can really say is that densities in the extremely early universe, just “after” the “bang”, were extremely high. The problem with thinking of it all starting from a point is that it suggests that there is a center that everything is rushing away from. In fact, however, the Big Bang happened everywhere. If you must think about it as that point, that point has itself expanded to the whole infinite Universe… and we, right here, are within that point, as is a distant galaxy a billion light-years away. In the Big Bang theory, the Universe has no center. Any point is as good as any other, and indeed, an observer looking from any point would see things around her as if she were at the center of the expansion! Which leads me to the T-shirt I want to have made one day. On the front: “Yes, in fact, I am at the center of the Universe!…” ; on the back: “…but so are you.”
We know little or nothing about the moment of “Bang” itself.
This is perhaps the most egregious. The Big Bang theory is tremendously successful, tremendously well supported by observations, yet… the thing that the theory is named after is something that the theory as it exists right now really can’t address. Sad, huh? What the Big Bang theory tells us is that the Universe evolved from a hot and dense state to what we have now. There are various different epochs we have been able to probe using different techniques, and the whole picture hangs together very well using these very different techniques. Right now, we have the expansion of the Universe. At a moment a few hundred thousand years after the “beginning”, we have the cosmic microwave background observed by the WMAP satellite and large numbers of other ground, balloon-borne, and space experiments. A few minutes after the Big Bang, we have the time when the Universe was so hot and dense that nuclear reactions routinely happened, at which the primordial elements were created… in proportions that match what we observe. And, finally, a tiny fraction of a second after the beginning, we have Inflation, which isn’t as well supported as those other bits, but which does explain a lot and even has stood up to one test from recent WMAP results. (Indeed, many people, myself included, are attracted to the notion of calling the end of Inflation “the beginning”, as subtracting that tiny fraction of a second from any later epoch won’t make any difference in the time we quote, and to talk about things before that you need Physics we don’t know how to do.)
All those different epochs, tied together by one expanding Universe theory that started from an extremely hot and dense state, all supported by a vast array of different observations. Pretty cool.
But.
The classical Big Bang– what you get if you just look at the Universe with Einstein’s General Relativity, ignoring Quantum Mechanics– gives a well-defined “beginning”, that time we call t=0 and measure all the other times from. That beginning is the singularity, where things diverge, where densities go infinite. “What caused it” is something we don’t even know how to address (unless you’re a string theorist talking about branes, but that’s a whole nuther issue).
The problem is, the classical Big Bang ignores Quantum Mechanics, which is a bad idea: Quantum Mechanics is another extremely well-supported theory in Physics we know to be right. It’s the theory of the very small (speaking very roughly). At some time a tiny fraction after the moment of the classical Big Bang, the Universe got large enough that it became reasonable to do your Quantum Mechanics (e.g. for the nuclear reactions a few minutes after the beginning) without worrying about gravity, and to do General Relativity (which describes gravity) without worrying about Quantum Mechanics. However, before that moment, you have to worry about both at once. Here’s the rub: the two theories don’t work together. At the moment, we don’t have a working theory that can handle gravity and quantum mechanics at the same time, although the string theorists keep telling us that they’re working on it.
What the previous paragraph says is that our extremely successful physical theories are incapable of predicting what happened during the first tiny time interval after the moment of the classical big bang. Indeed, if you think about it in reverse– start now and go back in time– we reach an early period before which we can say almost nothing. We can go back through the present day expansion, through the epoch when galaxies first formed, through the epoch when the Universe was a plasma and the Cosmic Microwave Background was emitted, through the epoch when the elements formed, through the epoch when quarks and gluons were all mushed about in one big continuous mess, to Inflation… but we can’t go before that.
We don’t know what happened before that. Was there even a “t=0″ beginning? Dunno. Was there really a singularity? Dunno. What happened before Inflation? Dunno. What was the deal with the actual Big Bang itself? Dunno.
Kind of sad that this extremely successful, extremely well-tested theory, a theory so good that we can call it right and true, doesn’t really address the moment it seems to be named after.
That’s why, marketing reasons aside, Big Bang is a terrible name for that theory. But I sure as hell can’t come up with a better name that doesn’t violate all sorts of marketing, so I guess we’re stuck with it.
1 Comment
Posted in Cosmology, Physics & Astronomy, Science |
This morning in Second Life : Comets Crashing Into Jupiter
Posted on August 8th, 2009 — permalink
I’ll be giving a public-outreach astronomy talk as part of MICA this morning at 10AM PDT in Second Life entitled “Comets Crashing Into Jupiter”.
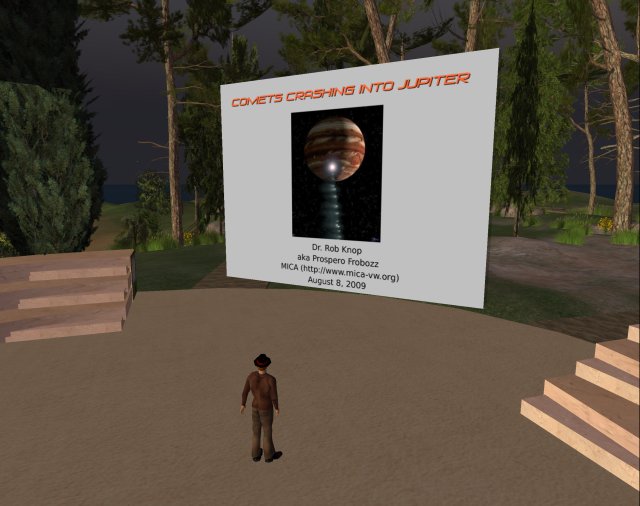
A few weeks ago, an amateur astronomer spotted a scar on Jupiter, which was later confirmed to be the result of an impact of a comet fragment with the giant planet. This is not the first time we’ve observed comets smacking into Jupiter; in 1994, comet Shoemaker-Levy 9 collided with Jupiter after having been torn into several fragmets by an earlier pass with the planet, leaving scars on Jupiter over the course of a week. In this talk, I’ll describe the gravitational interactions that lead to these comet collisions, as well as what we may be able to learn about Jupiter as a result of these collisions.
To keep track of what popular talks are upcoming in MICA, see our Upcoming Public Events Page.
Comments Off
Posted in Physics & Astronomy, Science, Second Life, Second Life Events |
How much Dark Matter do you hold in your hands?
Posted on July 9th, 2009 — permalink
Via a Cosmic Variance blog post, I found this astro-ph paper by Catena and Ulio that claims the local density of Dark Matter is 0.39 GeV/cm3.
What does this mean?
Well, first, you may object that GeV is a unit of energy, so really what we’ve got here is an energy density of Dark Matter, not what most of us mean when we say “density” all by itself (which is mass density). Physicists, however, know that converting between energy and mass is so easy (via E=mc2) that they will very often, especially when talking about fundamental particles, cite masses in energy units. Divide by the speed of light squared to figure out that this is a density of 6.9×10-25 grams per cubic centimeter.
Second, “local” means “in our area of the Galaxy”. Dark Matter clumps on scales of hundreds of light-years, at the smallest, so if you’re looking on a scale smaller than that, it’s going to look smooth. It’s similar to our atmosphere; if you climb a mountain and get five miles above sea level, the air will be noticably thinner. But, if you go up just a few meters, you’re not going to notice any difference in the density of air.
What is the density of air? Well, if you believe Wikipedia’s Answer (and you should in this case), it’s about 0.075 lbm/ft3… or, in more reasonable units, 0.0012 grams per cubic centimeter. (That’s at room temperature and standard sea level pressure.)
And, while we’re at it: the density of Dark Energy in the Universe is about 75% of the critical density, the critical density being 3H02/8πG, or 9.7×10-30 grams per cubic centimeter (for a Hubble constant of 72 km/s/Mpc).
Right.
So let’s put this all together. Cup your hands. That makes a box roughly 5cm on a side, or 125 cubic centimeters in size. In your hands, you are holding:
- 0.15 grams of air
- 8.7×10-23 grams of Dark Matter
- 9.1×10-28 grams equivalent of Dark Energy
Strictly speaking, “holding” isn’t the right term, because the Dark Matter particles are passing right through your hands as if they weren’t there… but at any one moment, that’s how much Dark Matter is on average within the box you make by cupping your hands. (Air is coming in and out as well, because you probably have cracks in the box you make, but it doesn’t pass through your hands.) And, it’s hard to say whether Dark Energy is passing through your hands, or is a property of the vacuum and as such it is just how much energy density is there as a result of the volume you’ve cupped, but you get my drift.
Oh, and I suppose I should mention that every second, about 140 million neutrinos from the Sun pass through your cupped hands (based on the neutrino flux cited at this page from HyperPhysics). However, they’re going so bloody fast (very close to the speed of light) that at any moment, there’s only about a 2% chance that one of those neutrinos is right then within your hands. (A similar consideration is probably true with Dark Matter particles, but I’d need to know the mass of said Dark Matter particles (nobody does), and I’d need to know their velocity (there are estimates, but I haven’t tracked them down) to actually give you numbers.)
1 Comment
Posted in Physics & Astronomy, Science |
“Superluminal Jets in Quasars” — Saturday in Second Life, 10AM PDT
Posted on June 26th, 2009 — permalink
Tomorrow (Saturday) in Second Life I’ll be giving a public talk about the relativistic jets we see emerging from the cores of active galactic nuclei, and in particular I’ll explain how it can be that some of them are apparently moving faster than the speed of light…!
The talk will be at the MICA Large Ampitheater in Second Life at 10AM PDT (Noon CDT, 1PM EDT). Remember, a Second Life account is free! You can join via the SciLands registration portal– if you do, that will take you to the SciLands orientation experience. (StellaNova, the in-world home of MICA, is part of the SciLands.)
Comments Off
Posted in Physics & Astronomy, Science, Second Life, Second Life Events |
“The Speed of Spacetime”, not the “Speed of Light”
Posted on June 25th, 2009 — permalink
That thing that we call the “Speed of Light” — which is, numerically, 3×108 meters per second, or, more elegantly, one light-year per year — is in fact not a property of light itself. Rather, it is a property of spacetime.
Relativity tells us that there is a fundamental speed scale to the Universe. That speed is the “speed of light”. Why it has this value, we don’t know; as far as relativity is concerned, it’s an initial condition, something that just is a property of our particular Universe. This speed scale can also be seen as the natural conversion between space units and time units — the natural scale of our Universe is such that there are 3×108 meters in one second. This speed scale is necessary to talk about “spacetime” instead of space and time as separate things. And, according to the theory of Relativity, they are not separate things. Depending on how one person is moving relative to another person, what one person considers to be pure time will be a mixture of space and time to the other person. How mixed it is depends on their relative velocity.
Relativity has an ultimate speed limit, a speed that nobody with non-zero mass can attain, but can only approach. Nobody is moving faster than the speed of light relative to anybody else. As they accelerate more and more, as they gain more and more momentum and energy, they get closer and closer to the speed of light, but they never quite get there. Meanwhile, particles with zero mass always move at exactly the speed of light (in the absence of a medium with which they interact). Photons happen to be particles of zero mass, but they aren’t the only ones. Gravitons also move at the speed of light– although we have yet to individually detect Gravitons. We have, however, detected (indirectly) gravitational waves, and those (just like light waves) move at the speed of light. That is, the speed of gravity is the same as the speed of light. And, this is no concincidence. In fact, both of them are moving at something more fundamental, the basic speed scale of spacetime.
For this reason, calling this speed limit “the speed of spacetime” would be more accurate than calling it the speed of light– although I grant you that that might be more confusing, and for general use “speed of light” is probably a better term.
Comments Off
Posted in Physics & Astronomy, Science |
The mysterious too-dim supernova discovered by a 14-year-old
Posted on June 13th, 2009 — permalink
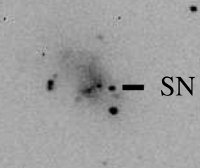
Supernova SN 2008ha is a particularly interesting supernova, for two reasons. First, because it was discovered by a 14-year old New York student. I would be very interested to know more about how Caroline Moore discovered this supernova. Was she working with a school project? With a professional astronomer’s “crowd sourced astronomy” project? What data did she use? Did she do it herself in her backyard with an amateur telescope and a CCD? Inquiring minds want to know, and if somebody has more information about this, please post a link!
The other thing that’s interesting about this supernova is just how dim it is. In America, of course, it’s strange to say that. We’re always interested in the biggest and baddest. The most distant! The brightest! The most energetic! The most luminous! But, just like “intermediate mass black holes”, this supernova seems to fall into a gap that makes it difficult to understand exactly what it is. Not that there aren’t things that it could be— but the problem is, if anything, there are too many things it could be!
The press release I link to above describes it as bridging the gap between novae and supernovae, but that may not really be an accurate description. Both novae and thermonuclear supernovae (but not core-collapse supernovae) come from a similar progenitor system. A white dwarf star— a star 1.4 times the mass of the Sun, but only the size of the Earth— pulls matter off of a companion star, probably a red giant. The matter builds up on the white dwarf, until one of two things happen. In a nova, the outer layers of the star get dense enough to trigger nuclear fusion in just those outer layers. An explosion ensues, and we can see it as a nova. The same binary system can produce a nova more than once. In a supernova, the white dwarf star reaches a critical mass known as the Chandrasaekar mass, and the entire 1.4 solar masses of star blows itself away in a tremendous thermonuclear explosion.
Thermonuclear supernovae are important not just because of what they represent in the potential life cycle of a star, but also because every time they explode, they release about the same amount of energy— The Chandrasaekar Mass is pretty much the same for every star. This has allowed them to be used as “standard candles” in measuring the expansion rate of the Universe, and it was observations of these objects that led us to the discovery that the expansion of the Universe is accelerating.
SN 2008ha was roughly 100 times dimmer than a typical thermonuclear supernova. This wasn’t because it was behind dust; the velocities measured in the expanding gasses were also much lower than is typically observed in a supernova. However, it’s much too luminous to be a mere nova. So what is it? The possibility suggested by the press release is that it really is something between a nova and a supernova: more than the outer layers of the star exploded, but it wasn’t the massive explosion of the entire star. However, if you look at the submitted paper describing follow-up observations of this event, we’re not really sure just what it was. The paper seems to be leaning towards it probably coming from a white, but it’s uncertain as to whether the explosion mechanism was core-collapse or thermonuclear. If the mechanism was core-collapse, then this is much like a very low-luminosity version of a traditional core-collapse supernova that signifies the end of the line for a massive star. In this case, it would be a star that was just barely massive enough to go supernova, and indeed, the supernova might have happened after the star shed its outer layers and left behind a white dwarf. No doubt that further work— observational on this event, and hopefully on more discoveries like this one, as well as theoretical— will be necessary to have a better idea of what really happened.
These events are probably quite rare, indeed probably rarer than normal thermonuclear supernovae (which happen, very roughly, about once per 500 years per galaxy). However, the fact that we only know of one right now shouldn’t be interpreted to mean that it’s a singular event. Thermonuclear supernovae are rare, but they’re bright, so we can see them in distant galaxies, and we’ve developed techniques to find them in great numbers. These dimmer events will be much harder to find.
It’s always exciting when something new, or at least something that shows a new wrinkle, is found in nature.
2 Comments
Tags: supernova supernovae
Posted in Physics & Astronomy, Science |
Wikimedia Commons Pictures of the Year
Posted on June 3rd, 2009 — permalink
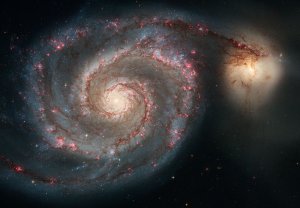
Wikimedia Commons is one of those web resources everybody should be aware of. It’s an archive of sounds, animations, and images that are available for your reuse under some sort of Creative Commons licencing (or are public domain). Among other things, you find the images embedded in Wikipedia here.
A link from Boing Boing led me to the 2008 Wikimedia Commons picture of the year. There are quite a number of pictures selected out on that page. To be sure the highest voted pictures are great, but my two favorites were not the top two images.
One was the one I’ve included here— but, of course, it’s an image I’m already familiar with, and one that could be described by the title of this blog! It’s the famous Whirlpool Galaxy, known to amateur astronomers as M51 and to professional astronomers by any number of catalog names (although usually as M51). The galaxy has very strong, very well-defined spiral arms, making it a classic “grand design” spiral. The fact that the spiral arms are so well-defiend is probably as a result of the interaction with the smaller galaxy that’s off to the right in the image. A self-gravitating, rotating disk naturally rings in a spiral density wave pattern when you disturb it, just like the smooth surface of water “rings” in concentric circular waves to a point disturbance (e.g. throwing a rock into the water), or in parallel ripple waves to a plane disturbance (e.g. a steady wind blowing across the water). (You’re probably more familiar with the term “ringing” when applied to the vibrations that occur in a bell when it is disturbed.) The gravitational interaction with the companion galaxy is almost certainly the disturbance causing such a well-defined spiral pattern in the primary galaxy. This particular image of M51 was taken with the Hubble Space Telescope.
I think my favorite image of the set, though, is Reflection in a soap bubble. It’s a beautiful photograph, and it has a sort of Escheresque quality to it. (Exploring the geometry of these sorts of reflections was the sort of thing Escher loved to do.) This is a photograph by Mila Zinkova, and is available under the GNU Free Documentation License. (I don’t believe that I need to make this post available under the GNU FDL simply by including the image, but I could be wrong.)
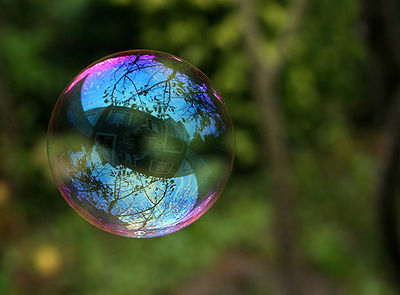
3 Comments
Posted in Free Culture, Physics & Astronomy, Science |
Shakepseare & Relativity in-world this weekend
Posted on May 28th, 2009 — permalink
I’m going to be busy this weekend with a public outreach talk and some Shakespeare performances.
Saturday morning at 10AM SLT/PDT, I’ll be giving a talk entitled Time dilation and simultaneity in Special Relativity. This is part of the regular “Dr. Knop Talks Astronomy” public-outreach talk series given by MICA. If you’re curious about just why it is that moving clocks run slow in special relativity, drop by and I’ll explain it. You don’t need any math to understand the basics; with just early high-school algebra (the Pythagorean Theorem), you can even understand the equation for how slow clocks run. The talk will be at the MICA Large Amphitheater (StellaNova (213, 210, 32)).
Then, Saturday and Sunday from 4PM-5PM, Avatar Repertory Theater will be performing scenes from several Shakespeare plays in our “Shakespeare at the Pavilion” performance, in association with the San Diego City sim. We’ll do scenes from Twelfth Night, Julius Caesar, Hamlet, As You Like It, and A Midsummer Night’s Dream. See the A.R.T. site for more information.
I remind everybody that a Second Life account is free. Here is one place you can register for an account— that’s the registration portal for the SciLands, a contiguous group of regions of which MICA is a member. That will take you to the SciLands’ orientation spot. Both of the events above will use Second Life Voice, but you don’t need a microphone or a headset; you’ll just need your standard computer speakers to hear what’s going on. There are Second Life viewer applications for Linux, MacOs, and Windows.
Comments Off
Tags: shakespeare relativity mica stellanova second life 2ndl
Posted in Physics & Astronomy, Science, Second Life, Second Life Events |
When Stars Collide
Posted on May 26th, 2009 — permalink
When I’ve given talks about colliding galaxies, I always start out by pointing that that the stars within a galaxy basically never collide with each other. This is, of course, an oversimplification….
You may have seen a “scale model” of the Solar System. It gives you a sense of how amazingly spread out things are… especially after you’ve made the hike to get to Saturn, never mind Uranus, Neptune, or the Kuiper Belt. But you never see a scale model of the nearby stars. Why? because things are even more amazingly spread out. Suppose I wanted to make such a scale model, and, here in Nashville, TN, I used a tennis ball to represent the Sun. To represent the nearest star, α Centauri, I’d need another tennis ball that is in New York City. Stars are really, really far apart from each other. Our galaxy is mostly empty space. (Well, actually it’s mostly dark matter, but within the disk of the galaxy, the mass density is more stars than dark matter. And, between the stars, there is interstellar gas, but the density of that gas is much lower than the density of gas in the best vacuum chamber we’ve built on Earth.)
However, there are places where this isn’t strictly true. We see some stars in globular clusters that are more massive than stars that have any business still being around in that globular cluster. (That is, globular cluster stars were all formed 11-13 billion years ago. Stars with higher mass have shorter lifetimes, so any star above a certain mass cutoff isn’t seen in a globular cluster.) One very likely explanation for this is that these stars are the result of a merger between two lower-mass stars.
It may also be possible in some very rich young clusters of stars that stars may collide with each other before the cluster disperses. The black hole at the center of our galaxy may very rarely “eat” a star. (No, we haven’t seen this happen.)
Last week, Jamie Lombardi of Allegheny college gave a seminar in Second Life as part of the MICA professional seminar series entitled “The Hydrodynamics of Runaway Collisions.” At the very beginning of the talk, he had to clarify that he was in fact talking about colliding stars. I give a lot of public-outreach astronomy talks for MICA (a different series, obviously, from the serious of professional seminars), and I have given a couple of talks about colliding galaxies. My own history as an astronomer leads me to think “collisions” to mean “between galaxies”– and the hydrodynamics, then, must be referring to the gas processes that might, say, feed an AGN.
However, cool things can happen when three stars have a close enough pass that their envelopes start to spill on to each other. Sometimes, two of the stars will merge (while the third one in the collision is ejected). Some of the gas is lost, but much of it stays behind in a more massive star. The timescale for these collisions is short enough that the stars will not evolve appreciably during the collision, so this may well be a way that you can make a star that’s more massive than what would naturally form out of star formation nowadays— especially if you can chain together several collisions. What Jamie was talking about, of course, probably only applies in very young, very rich clusters. The globular clusters in our Galaxy today are too old to have the kind of massive stars that Jamie was focusing on in his talk, and most of the star forming clusters nowadays have several orders of magnitude fewer stars than globular clusters do. But, globular clusters were once young….
Comments Off
Posted in Physics & Astronomy, Science, Second Life Events |
This Saturday at 10AM PDT in SL : “How We Know Dark Matter Exists”
Posted on April 29th, 2009 — permalink
As part of my regular Dr. Knop Talks Astronomy series of public astronomy lectures in Second Life, this coming Saturday I’ll be talking about how we know that Dark Matter really exists.
The talk will be at the Large Ampitheater on StellaNova. Note that a Second Life account is completely free! You can register for an account here. This is the “SciLands” entry portal, which will put you at the SciLands own orientation island. (StellaNova, the sim of MICA, is part of the SciLands.)
Here’s a description of the talk:
Modern cosmology tells us that the majority of the Universe is made up of stuff whose nature is largely unknown to us. Two thirds of it is Dark Energy; most of the rest is Dark Matter, the subject of this talk. Dark Matter interacts with normal matter through gravity, but otherwise it interacts hardly at all. Yet, we have very high confidence that this mysterious Dark Matter really does exist. Because it doesn’t interact with light, we haven’t seen it glowing, nor have we observed it absorbing background light as we’ve seen with dust clouds. All of the evidence we have for Dark Matter comes from its gravitational interaction with other matter, and with light. Yet, this evidence is extremely compelling. In this talk, I will attempt to convince you that there is no reasonable doubt that Dark Matter exists.
Comments Off
Posted in Cosmology, Physics & Astronomy, Science, Second Life, Second Life Events |